- Published:
- 15 July 2024
- Author:
- Dr Subarna Chakravorty
- Read time:
- 6 Mins
This article reports on the development of gene therapy treatment for sickle cell and thalassaemia.
In its April 2024 issue, the American magazine Time named Professor Stuart Orkin of Harvard University as 1 of the 100 most influential people in the world. This acknowledges his pioneering work in understanding globin gene expression that has led to the first-in-world approval of gene-editing in achieving cure in sickle cell disease (SCD) and thalassaemia.
Within the last few months, both the Food and Drug Administration (FDA) in the USA and the UK’s Medicines and Healthcare products Regulatory Agency (MHRA) have approved exagamglogene autotemcel (exa-cel), a cellular product that uses the new gene-editing technique CRISPR-Cas9 to achieve a functional cure. The historic significance of this approval for human use, occurring only 3 years after Professors Jennifer Doudna and Emmanuelle Charpentier were awarded the Nobel Prize in chemistry for its discovery,1 cannot be overstated. It reflects the enormous scientific curiosity for understanding basic biological processes and harnessing this knowledge for application in science and medicine2 and is truly seismic in importance.
SCD affects an estimated 7.4 million people worldwide, and globally ranks 12th highest in all-cause mortality in childhood.3 It is characterised by a point mutation in the gene encoding β-globin. The mutant haemoglobin tends to polymerise in hypoxic conditions, giving rise to rheological changes to the red blood cell. This leads to haemolysis, vaso-occlusion, ischaemia-reperfusion and its consequent clinical features of severe episodic pain, organ damage and reduced life expectancy.4 β-thalassaemia, another severe genetic condition affecting β-globin, results in ineffective erythropoiesis, severe anaemia, iron overload and untimely death. Thalassaemia also affects many millions worldwide, and can be fatal if transfusions and iron chelation are not regularly provided.5
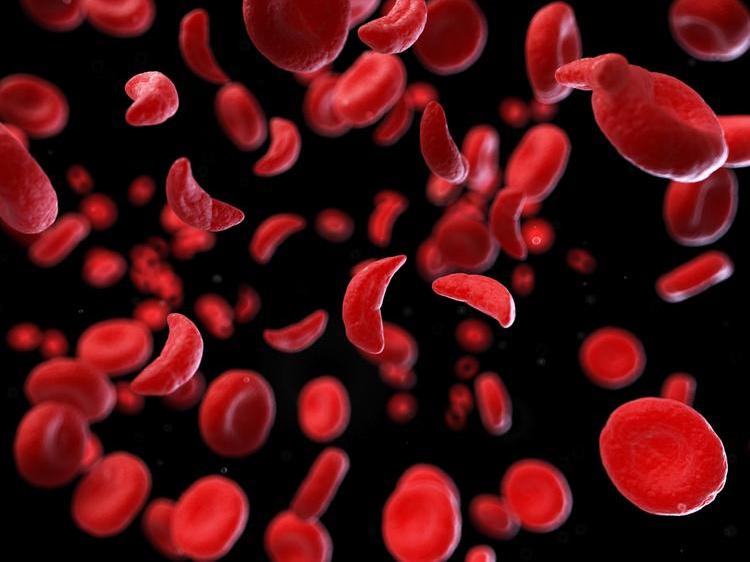
Despite the well-described pathophysiology, SCD patients have a huge variability in disease manifestation. Over several decades, haemoglobin (Hb) F has emerged as a key modifier of disease severity.6 It causes significant delays in the lag time needed to form HbS polymers, thereby reducing hypoxia-induced polymerisation in the microcirculation.7 Naturally occurring mutations in the β-globin gene cluster result in persistent expression of HbF (HPFH), and results in almost complete abrogation of pathological consequences of sickle cell anaemia if co-inherited with the sickle mutation.8
This finding has prompted the use of the HbF inducer hydroxycarbamide as a key therapeutic agent in the management of SCD.9 About 15 years ago, genome-wide association studies identified sequence variants in a trans-acting gene BCL11A on chromosome 2 that influence HbF levels.10 Further molecular dissection, pioneered in Professor Orkin’s laboratory and replicated by many others, established the direct role of BCL11A in globin gene regulation, acting as a repressor of HbF expression in adult erythropoiesis.11 Increased HbF expression also causes amelioration of β-thalassaemia symptoms,12 and can achieve transfusion independence if expressed in adequate amounts. Thus, disrupting the repression of BCL11A in patients with SCD and thalassaemia by gene-editing techniques has generated significant scientific interest.
Manipulating the haemopoietic stem cell genome in SCD and thalassaemia to achieve cure has been investigated for decades. One successful technique has been tried in animals and humans, in which the entire β-globin gene, pre-programmed to have one or more anti-sickling mutations and most of its upstream regulatory elements, is incorporated into a lentiviral vector (LVV) and transfected into haemopoietic stem cells.13
Using this technology, 2 gene insertion products, lovotibeglogene autotemcel (lovo-cel) and betibeglogene autotemcel, have recently been approved by the FDA to treat SCD14 and β-thalassaemia.15 Another gene-editing technology targeting the BCL11a gene, using post-transcriptional silencing by short hairpin RNA has demonstrated clinical efficacy in a Phase 1/2 study.16 Several other approaches to improve HbF induction have been investigated. These have included CRISPR-Cas9 targeted disruption of the promoter regions of γ-globin genes HBG1 and HBG2.17
Although promising, several challenges still remain in the use of these new techniques. All of these procedures require an autologous stem cell harvest, followed by ex vivo gene insertion or gene editing, and returning the engineered stem cells to the patient following host myeloablation. This has mostly been done by using the alkylating agent busulfan, resulting in significant toxicities involving myelosuppression, febrile neutropaenia and risk of infertility, to name a few. Although other myeloablative techniques, including monoclonal antibodies18, are currently under investigation, none have reached advanced-phase clinical trials.
Gene addition techniques are potentially subject to insertional mutagenesis, a phenomenon that can be reduced when using CRISPR-Cas9 techniques. Recent reports of myelodysplasia (MDS) and acute myeloid leukaemia (AML) in sickle cell patients undergoing treatment with lovo-cel were subject to intense investigations by the study team.19 Although no direct causation by the inserted gene was proven in the aetiology of the MDS/AML, it has brought to light the importance of careful patient selection and post-therapy monitoring. Additionally, genotoxicity of off-target double-stranded breaks induced by CRISPR-Cas9 has led to concerns about future oncogenesis. Newer, more precise gene engineering methods,20 using base-editing or prime-editing, could provide more reassurance towards clinical safety.
The next frontier in the search for technologies that provide cure by gene therapy in SCD and thalassaemia is to avoid the need for ex vivo gene manipulation altogether, and undertake in vivo gene engineering instead. Many investigators are now working on novel methods to deliver the gene therapy medicinal products directly to patients without the need for autologous harvest or myeloablation.21 Although it may sound far-fetched, scientific methods needed to achieve gene correction in vivo are advancing at a fierce pace and we envisage this will become available for human trials within years.
In March 2024, the UK’s National Institute for Health and Care Excellence (NICE) published a draft decision not to approve exa-cel for NHS funding but, at the time of writing, its final decision, following further consultation, has not been published and is still awaited.
Patients and physicians have long lamented the paucity of effective therapy in these conditions, and access to such treatment will be greatly welcomed by the community. However, there needs to be careful consideration in patient selection, pre-procedural assessments, and long-term post-procedural follow-up. Patients will also need to be aware of the uncertainties of the long-term outcome of novel therapies.
Despite the remarkable scientific advancements in gene therapy in SCD and thalassaemia, costs remain prohibitive, making these treatments virtually inaccessible to the vast majority of patients with these conditions. Even in wealthy countries, upfront 7-figure payments, as in the case of lovo-cel, which was approved in the USA in 2023 for $3.28 million, will be challenging for insurance companies to pay.
Publicly funded healthcare systems in Europe will be significantly stretched unless an acceptable pricing is agreed. To make gene-manipulated products available to patients in low-income countries, innovative and frugal approaches are being investigated. These approaches include the use of a decentralised manufacturing process for anti-CD19-CAR-T cells using a fully automated closed system (CliniMACS Prodigy) in India.22
David Weatherall, prominent in the field of haemoglobinopathy, presciently wrote in 1984 that ‘the description of an efficient method for transfecting haematopoietic stem cells offers major encouragement to those who believe that the ultimate goal of clinical genetics is gene replacement therapy, rather than termination of pregnancy’.23 For those believing in health equity, this may be taken further to include all patients with these life-limiting genetic diseases, regardless of where they live.
References available on our website.
Return to the July 2024 Bulletin