- Published:
- 20 January 2025
- Author:
- Tim Lang
- Read time:
- 9 Mins
In this article, Tim Lang of Newcastle-upon-Tyne NHS Foundation Trust explains the requirements of paediatric patients in laboratory medicine, from diagnosing and identifying risk factors to developing adequate testing and sampling technologies.
Paediatric laboratory medicine (PLM) must continue to expand to meet the needs of this special patient group and evolve for the future. PLM has been at the forefront of the development and adoption of emerging technologies that ensure that the patient’s journey through early life to adulthood allows them to reach their full health potential. Any laboratory service that supports a healthcare facility where a neonate, infant, child or adolescent may attend must be aware of the specific requirements and issues associated with this patient group. Expanding access to appropriate laboratory services to support the paediatric patient group is essential, especially to populations excluded due to socioeconomic or geographical reasons.
Over the past 10 years, there has been a rapid adoption of emerging technologies, including genomics and artificial intelligence/machine learning, to address some of the longstanding diagnostic challenges that this population has faced. However, laboratory services and diagnostics manufacturers also need to remain aware of issues such as sample volume, assay calibration, resource availability and the emergence of new and old diseases.1
During the first weeks of life, PLM has an important role in supporting the transition from foetal development to self-regulating homeostasis, in addition to identifying pre-symptomatic but treatable conditions through newborn blood spot screening (NBS). There have been many advances in NBS recently in the UK, with the planned addition of tyrosinaemia type 1 expected and an in-service evaluation in progress for severe combined immunodeficiency. The machine-learning-based technique of computer vision has been developed to support the process of accurately identifying adequate newborn blood spots and shows promise.2 Given the improved accessibility and affordability of whole genome sequencing, Genomics England has embarked on the Generation Study, which plans to sequence the genomes of 100,000 babies to improve the ability to diagnose and treat over 200 genetic conditions.
During the first few days of life, the neonate is exposed to several conditions or risks that may cause potential short- and long-term harm if not identified immediately. PLM’s role is to support the clinical team in detecting and accurately quantifying biomarkers, such as bilirubin and glucose, to enable appropriate management. Recognising and quantifying neonatal jaundice remains a challenge, even for an established laboratory service, given the known biases of the common clinical chemistry analyser methods.3 While transcutaneous bilirubinometers are now established in practice, providing a non-invasive method of quantitating bilirubin concentrations, they still lack the necessary analytical performance at the concentrations required for active treatment, such as phototherapy or exchange transfusion.
Future use of biosensors linked to smartphone technology may provide an affordable and accessible solution in lower- and middle-income countries where access to laboratory-standard analysis may not be readily available in a timely manner. However, education is also essential to ensure that the associated risks in neonates are identified so that treatments can be initiated promptly.
This is also the case in identifying infants at risk of neonatal hypoglycaemia, where the symptoms can be non-specific or even silent. The actual glucose concentration at which harm is done remains undetermined; however, to prevent harm, action limits have been proposed to ensure appropriate treatment is initiated, such as glucose gels as advised by the Sugar Babies Study in New Zealand. Monitoring biomarkers in this specific population often requires repeated phlebotomy, which can potentially result in iatrogenic anaemia.4,5
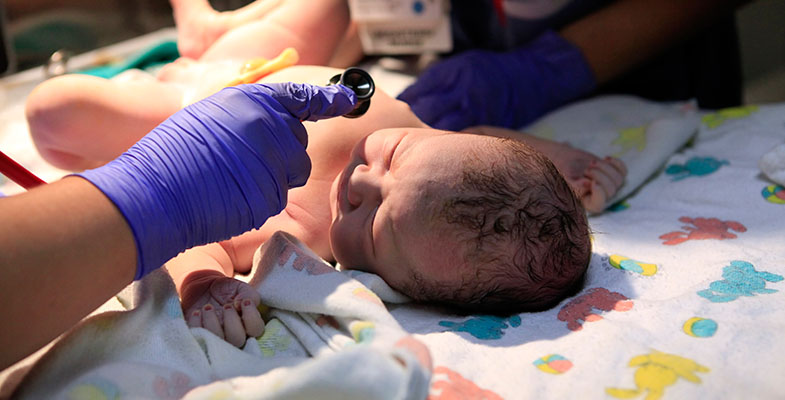
The majority of laboratory-based multi-channel analysers are designed for the adult population, so there is normally a requirement for volumes greater than 100 µl to perform a set of tests. While the drive to be more sustainable and reduce waste has reduced the volume required for these analysers, development of novel techniques that require a minimal amount of blood is still needed. Point-of-care testing (POCT) solutions have addressed some of these issues, but few have been specifically designed with the neonatal/paediatric population in mind to meet the required performance criteria for practice.
A development using an existing POCT solution originally designed for adult use is showing promise in the monitoring of neonatal glucose concentrations using continuous glucose monitoring sensors. This technology is being used to address some of the uncertainty of neonatal glucose homeostasis and is being coupled with glucose infusions and insulin pumps to prevent hypoglycaemia in at-risk infants.6
Sepsis is another important clinical episode that neonates and infants may be exposed to, and which requires immediate identification and management. Machine learning is being used to identify the best diagnostic biomarkers/clinical signs to identify sepsis and those at risk of it.7 With the improvements in, and increased access to, benchtop genomic analysers, clinicians can now identify the pathogenic organisms in a significantly shorter timeframe at the bedside and also avoid potentially unnecessary antibiotic use, having learned from the experience of the COVID-19 pandemic.
Most laboratories supporting a neonatal or paediatric population are based in hospitals serving a predominantly adult population, including some regional specialist children’s hospitals. In most cases, paediatric samples need to be dealt with differently from adult samples, owing to the volume of sample available and how they are collected. Conversely, the majority of assays on the common multi-channel clinical chemistry and immunoassay analysers require a significant volume of blood that may not always be available owing to the size of the patient. Therefore, manufacturers must develop assays with smaller volume requirements but the same diagnostic accuracy.
In addition, there is a push to improve collection devices to ensure the best quality samples are obtained with the minimum of distress to the patient and that do not require the patient to attend the hospital for collection. For example, Capitainers, a micro-sampling device, are being used to collect samples for phenylalanine monitoring in some patients with phenylketonuria without the need for clinic attendance. Alternatively, different matrices – ideally those collected non-invasively – are being used to investigate conditions which have traditionally used blood samples.
Emerging technologies have allowed matrices such as saliva, faeces, hair and sweat to be used to address a variety of conditions, including malaria detection, heavy metal poisoning and pre-natal drug exposure. Similarly, microassays that can provide accurate and rapid diagnostic answers continue to be developed that require even smaller volumes (<10–100 uL).
Sample integrity and pre-analytic artifacts are also important when managing paediatric samples. Long-standing procedures are beginning to be challenged so that potentially lifesaving management decisions are not delayed. For example, recent research from my own laboratory by Dr Ann Bowron and Dr Gavin Mercer-Smith has shown that samples for ammonia no longer need to be collected or transported on ice.8
From the post-analytical perspective, it is essential that the laboratory provides appropriate age-related reference ranges or cut-offs that are suitable for the paediatric population. Large-scale initiatives, such as the Canadian Laboratory Initiative on Pediatric Reference Intervals (CALIPER), using direct reference range production methodologies have become universally accepted.9 However, newer indirect methodologies are now being used that use data-mining processes to create more population-specific reference ranges where it may not be ethical or practical to use the direct approach of sampling individuals.
It is important to remember that the laboratory must assess the suitability of the reference range and validate it in its own population, as per Clinical and Laboratory Standards Institute guidance. An example to highlight this is using the CALIPER ranges to diagnose folate deficiency. These ranges were produced from a population where there was fortification of flour with folate; however, the UK currently does not routinely fortify flour with folate, so this range may overestimate the number of deficient individuals if using a higher lower limit of normal produced from a folate-replete population.
The last 5 years has seen the emergence of new and the re-emergence of old diseases impacting on PLM. The COVID-19 pandemic significantly impacted all laboratory services but especially screening programmes.10 The burden of later presentations of common childhood diseases resulted in potentially worse outcomes. Planning for staff contingencies, working differently and reagent availability were highlighted as areas to learn from. The laboratory also needs to be aware of the recurrence of old diseases due to poor nutrition, and cultural and lifestyle choices, and how diagnostic pathways may need to be reintroduced. For example, cases of scurvy (vitamin C deficiency) are being identified, requiring access to appropriate specialist analysis.
In summary, PLM continues to have an important role in supporting the patient journey from early life to adulthood through adopting new and emerging technologies. Through these advances, it will be best placed to ensure that appropriate laboratory services are accessible to all, including those from geographically remote and socially deprived areas, to ensure best patient outcomes.
References available on our website.
Return to January 2025 Bulletin